Solar Sail: Difference between revisions
THESABERLION (talk | contribs) |
No edit summary |
||
(38 intermediate revisions by 2 users not shown) | |||
Line 1: | Line 1: | ||
Solar sails, or more specifically solar photon sails, use the radiation pressure from the light emitted from the Sun to propel spacecraft through space without using any propellant. In an almost poetically similar manner to a sail sea ship. | Solar sails, or more specifically solar photon sails, use the radiation pressure from the light emitted from the Sun to propel spacecraft through space without using any propellant. | ||
In an almost poetically similar manner to a sail sea ship. | |||
Light is reflected from huge, extremely thin, reflective sheets made as light as possible, sometimes too light to survive any force much larger than the radiation pressure from the light. | |||
They can only be used in space. | |||
The “sails” are extended over some “rigging” structure that keeps the sail taut and attaches the payload to the solar sail so it can be carried away. | |||
Solar sails can be used for many different purposes, typically space maneuvers that require little thrust and are not significantly limited in duration. | |||
They can be used for station keeping, orbital maneuvering or interplanetary propulsion. | |||
They typically accelerate very slowly, like ion-engines. | |||
Some more optimized designs can pick up tremendous speeds given enough time and adequate materials and construction. | |||
==Introduction== | |||
Solar sails, also known as photon sails or light sails, are devices that can exploit an interesting quirk of relativity for propulsion. | |||
When a photon is absorbed on or reflected from a surface, it applies a force on it. | |||
This is denominated radiation pressure, and is typically a very tiny tiny value (did I say tiny?). | |||
So, if we deploy a very large reflective surface to reflect photons, that can also be made to be extremely thin and lightweight, it’s possible to produce a substantial force to push a spacecraft around so you can go to places, not unlike a sailing sea vessel. | |||
With the great advantage of not consuming any propellant while doing that, so you don’t have to carry any! | |||
This makes solar sails a propellantless propulsion system, and so we don’t have to worry about that pesky rocket equation. | |||
Somebody once said there is no free lunch, and with solar sails we can have a propellant-free propulsion, but typically solar sails don’t generate much force, putting them in the same category of low-thrust methods like ion-engines. | |||
Due to their tiny thrust, the sails must operate in space, handling inter-orbit transits and interplanetary trajectories. | |||
Somebody once said there is no free lunch, and with solar sails we can have a propellant-free propulsion, but typically solar sails don’t generate much force, putting them in the same category of low-thrust methods like ion-engines. Due to their tiny thrust, the sails must operate in space, handling inter-orbit transits and interplanetary trajectories. Most solar sails are too heavy to accelerate faster than the local gravity, and thus need to spiral in or out of gravity wells like low power electric thrusters. | Most solar sails are too heavy to accelerate faster than the local gravity, and thus need to spiral in or out of gravity wells like low power electric thrusters. | ||
Although a really lightweight high performance solar sail could perform direct injections into interplanetary space relatively quickly. And even float over a star or a planet, as in a statite. Allowing us to build all sorts of crazy systems and structures. | Although a really lightweight high performance solar sail could perform direct injections into interplanetary space relatively quickly. | ||
And even float over a star or a planet, as in a statite. | |||
Allowing us to build all sorts of crazy systems and structures. | |||
===Propulsion Performance=== | ===Propulsion Performance=== | ||
Performance of solar sails vary wildly among the several designs that have been proposed. The design performance presented here comes from the concept proposed by Kim Eric Drexler (yes, the nanotech guy) in his 1977 Master’s Thesis. It’s a very high performance design that has inspired the photon sail design seen in “Rocheworld” by Robert L. Forward. Figures here do not assume a payload, so the sail can be used as an Engine, like an ion thruster. | Performance of solar sails vary wildly among the several designs that have been proposed. | ||
The design performance presented here comes from the concept proposed by Kim Eric Drexler (yes, the nanotech guy) in his 1977 Master’s Thesis. | |||
It’s a very high performance design that has inspired the photon sail design seen in “Rocheworld” by Robert L. Forward. | |||
Figures here do not assume a payload, so the sail can be used as an Engine, like an ion thruster. | |||
{| class="wikitable" | {| class="wikitable" | ||
|+ | |+ | ||
|Name | |Name | ||
| | |Lattice Sailer | ||
|- | |- | ||
|Thrust | |Thrust | ||
| | |7.26 N | ||
|- | |- | ||
|Mass | |Mass | ||
| | |100 kg | ||
|- | |- | ||
|Efficiency | |Efficiency | ||
| | |80% | ||
|- | |- | ||
|Power Consumption | |Power Consumption | ||
| | |1.36 GW | ||
|- | |- | ||
|T/W | |T/W | ||
|0. | |0.0074 (Calculated) | ||
|- | |- | ||
|Energy Source (Fuel) | |Energy Source (Fuel) | ||
| | |Sun | ||
|- | |- | ||
|Propellant | |Propellant | ||
| | |Solar Photons | ||
|- | |- | ||
|Specific Power | |Specific Power | ||
| | |13.6 MW/kg (Calculated) | ||
|} | |} | ||
Conditions are also taken as about 1 AU from the Sun. Assuming a sail capable of reflecting 80% of the incoming light and pointed directly at the Sun. | Conditions are also taken as about 1 AU from the Sun. | ||
Assuming a sail capable of reflecting 80% of the incoming light, with an areal density <math>0.1 g/m^2</math>, an area of <math> 1 km^2</math> and pointed directly at the Sun. | |||
<references group="C"/> | <references group="C"/> | ||
==Design and Function== | ==Design and Function== | ||
As described by relativity, photons of light might not have mass in a classical sense, but they carry momentum. So when a photon hits a surface, and sometimes bounces off it (emitting photons also works, that’s how the photon drive works), we have an exchange of momentum, not unlike a ball hitting a wall. The sum of all the tiny, tiny exchanges of momentum with a ridiculously large number of photons exerts a pressure on the surface. | As described by relativity, photons of light might not have mass in a classical sense, but they carry momentum. | ||
So when a photon hits a surface, and sometimes bounces off it (emitting photons also works, that’s how the photon drive works), we have an exchange of momentum, not unlike a ball hitting a wall. | |||
The sum of all the tiny, tiny exchanges of momentum with a ridiculously large number of photons exerts a pressure on the surface. | |||
[inset diagram] | [inset diagram] | ||
We call that radiation pressure, and under normal conditions, it’s usually very weak. But nonetheless, this pressure created by light, applied over a large surface creates a force that can move a spacecraft. For a surface that is perfectly absorbing, the radiation pressure can be calculated by dividing the incident irradiance (usually measured in W/ | We call that radiation pressure, and under normal conditions, it’s usually very weak. | ||
But nonetheless, this pressure created by light, applied over a large surface creates a force that can move a spacecraft. | |||
For a surface that is perfectly absorbing, the radiation pressure can be calculated by dividing the incident irradiance (usually measured in <math>W/m^2</math>), by the speed of light c (in <math>m/s</math>): | |||
<math alt="radiation pressure">P_i = \frac{I_f}{c}</math> | |||
<math alt= | |||
When the surface reflects a photon, it gets an exchange of momentum when the photon comes, and also when it is reflected back. So we get twice the pressure! | When the surface reflects a photon, it gets an exchange of momentum when the photon comes, and also when it is reflected back. So we get twice the pressure! | ||
<math alt="radiation pressure with reflection">P_{ref} = 2 \times \frac{I_f}{c}</math> | |||
Using this formula, we can calculate that the radiation pressure around Earth’s orbit from sunlight, where the incident irradiance is about 1,360 W/ | Using this formula, we can calculate that the radiation pressure around Earth’s orbit from sunlight, where the incident irradiance is about <math alt="solar constant">1,360 W/m^2 </math> is: [solar constant value] | ||
<math alt="radiation pressure calculation at 1 AU">P_{ref} = 2 \times \frac{1,360 W/m^2}{c} = 9.07 {\mu}Pa </math> | |||
So the pressure is just a bit over 9 micropascals, that’s about the pressure due to the weight of a small ant, distributed over one entire square meter! It’s nuts! But in space, we don’t have air resistance, so even a small force, applied continuously over a long period of time can really add up. Even more so, if we use extremely large and thin sails, that are super lightweight! They don’t need to be very strong, as they don’t have to support their own weight in space. And can accumulate a large enough force to allow for reasonable fast accelerations. | So the pressure is just a bit over 9 micropascals, that’s about the pressure due to the weight of a small ant, distributed over one entire square meter! | ||
It’s nuts! | |||
But in space, we don’t have air resistance, so even a small force, applied continuously over a long period of time can really add up. | |||
Even more so, if we use extremely large and thin sails, that are super lightweight! | |||
They don’t need to be very strong, as they don’t have to support their own weight in space. | |||
And can accumulate a large enough force to allow for reasonable fast accelerations. | |||
Light sails can have many configurations. But basically they contain three components. The payload, which is usually the spacecraft you want to move around. Attached to the payload is the sail and a system of booms and rigging to keep the sail taut and keep the sail in the right position and orientation. | Light sails can have many configurations. | ||
But basically they contain three components. | |||
The payload, which is usually the spacecraft you want to move around. | |||
Attached to the payload is the sail and a system of booms and rigging to keep the sail taut and keep the sail in the right position and orientation. | |||
[ | [[Image:sail_diagram1.png|400px|thumb|left|Simple diagram of a solar sail.]] <br/> | ||
Because radiation pressure is so weak, we want to build the most lightweight sail possible so we can generate as much force as we can, without also getting too much extra mass to accelerate. So solar sails are made of extremely thin reflective films of a wide range of materials. From films of polymers like polyimide or polyesters covered in an extremely thin metal layer applied by vapor deposition (like those space blankets), to extremely thin films of metal that are just nanometers thick, to more exotic materials like extremely thin layered dielectrics materials. Even graphene has been proposed (and tested in the lab) as solar sail material. | Because radiation pressure is so weak, we want to build the most lightweight sail possible so we can generate as much force as we can, without also getting too much extra mass to accelerate. | ||
So solar sails are made of extremely thin reflective films of a wide range of materials. | |||
From films of polymers like polyimide or polyesters covered in an extremely thin metal layer applied by vapor deposition (like those space blankets), to extremely thin films of metal that are just nanometers thick, to more exotic materials like extremely thin layered dielectrics materials. | |||
Even graphene has been proposed (and tested in the lab) as solar sail material. | |||
But also, as important as the sail, the rigging should not be neglected. Several types of rigging have been proposed for solar sails, from extremely lightweight trusses and inflatable booms that support and keep the sail stretched, to designs that are supported by centrifugal forces created by spinning the whole solar sail, and having masses at the edge to keep the whole thing taut. To very speculative designs that bounce some of the incoming light inside the structure of the sail to keep it inflated. | But also, as important as the sail, the rigging should not be neglected. | ||
Several types of rigging have been proposed for solar sails, from extremely lightweight trusses and inflatable booms that support and keep the sail stretched, to designs that are supported by centrifugal forces created by spinning the whole solar sail, and having masses at the edge to keep the whole thing taut. | |||
To very speculative designs that bounce some of the incoming light inside the structure of the sail to keep it inflated. | |||
==Sail Configurations== | ==Sail Configurations== | ||
===Heliogyro=== | ===Heliogyro=== | ||
The Heliogyro is a 1967 concept for a new type of solar sail proposed by Richard H. MacNeal from the Astro Research Corporation. | |||
The vehicle employs long, narrow blades constructed from thin films that are rotated around the spacecraft bus in a manner similar to a helicopter rotor. | |||
Another important feature is the deployment process, simply done by unrolling the sail blades from spools. | |||
To keep the sail stretched and in a given attitude, the blades are spun around to create centrifugal force to maintain them under tension. | |||
In one of the 1967 designs, one concept of the Heliogyro uses two blades, which are narrow sheets of Mylar with a thin film of aluminum, to make them reflective. | |||
They are around <math>1.5</math> meters wide and <math>5.7</math> km long, very narrow and super long, with a thickness of about <math>6</math> micrometers. | |||
To stop the blades from curling over the width, the blades feature a small compression batten every 30.5 meters or so. | |||
[ | [[Image:heliogyro_control.jpg|thumb|left|Heliogyro control by adjusting the pitch of its blades.]] | ||
For maneuvering, a solar sail must be able to control how much sunlight it is reflecting, to control the thrust, but also be able to turn around and change its attitude. | |||
Especially if it is tacking. In the heliogyro, the blades are attached to the main spacecraft by spools, which can rotate around the blade's longest axis. | |||
If the heliogyro is pointing at the Sun, this allows the control of how much sunlight is being reflected. | |||
This works similarly to the collective control of a helicopter. | |||
To cause the spacecraft to turn around, the pitch of the blades is changed as the blade rotates around. | |||
In a similar way to cyclic pitch control, also from helicopters. | |||
That way, it is possible to apply force on just one side of the sail to make it turn around. | |||
Both controls can be combined to do all sorts of maneuvers. | |||
There have been few studies on heliogyros, but one of the most detailed was done in 1977 by the JPL for a mission for a rendezvous with Comet Halley. | |||
That design was even larger, with blades <math>8</math> meters wide and <math>7.5</math> km long. | |||
Although in the end they preferred a solar electric propulsion concept over an untested solar sail technology like the Heliogyro. | |||
[[Image:heliogyro_halley.jpg|thumb|left|Heliogyro rendezvous with Halley's Comet.]] | |||
More recently, there was a 1989 study by an MIT team for a mission to Mars. | |||
With a smaller Heliogyro, using 8 blades, 100 meters wide. | |||
More recently, in 2014, NASAA performed a study on a possible Heliogyro demonstration mission called HELIOS (High-Performance, Enabling, Low-Cost, Innovative, Operational Solar Sail). | |||
The demonstrator would use six blades that would be 4.5 meters wide and 220 meters long. | |||
[[Image:heliogyro_mars.png|thumb|Heliogyro concept over Mars.]] | |||
Because most Heliogyro concepts feature vehicles that are launched from Earth and then have to deploy their sails, they usually feature relatively "thick" Mylar sheets. | |||
With that kind of limitation, most Heliogyro are usually limited to accelerations of the order of <math>\sim 1.5 mm/s^2</math> when near Earth. | |||
It's no neck breaking acceleration, but in space, even that can build up. | |||
Just to get an idea, you can gain around one kilometer per second of delta-V in just 8 days with that. | |||
[[Image:helios.jpg|thumb|left| HELIOS deployment sequence.]] | |||
===The Lattice Sailer=== | |||
In 1979, Kim Eric Drexler (the big guy behind nanotechnology) received his M.S. with a Master’s thesis titled “Design of a High Performance Solar Sail System”, where he described the design of a very high performance solar sail based on ultrathin aluminum films and a centrifugally stabilized structure, that he called the “Lattice Sailer” due to its rigging system. Instead of using thin plastic films covered with reflective metallic coats, the Lattice sailer makes use of thin aluminum films, in the range of tens of nanometers thick, that would be manufactured in space (such films are way too delicate to survive a launch and a deployment). The sail would be built of many smaller triangular segments. Each with a thickness ranging from 15 to 100 nm, and would only weigh <math> 0.04-0.27 g/m^2 </math> , tens of times lighter than the metallized plastic films. The sheets of this metallic film are covered in a pattern of small cuts and wrinkles that can remove tension in small areas of the film to stop tears from propagating. | |||
[ | [[Image:lattice_sailer.png|thumb|Lattice Sailer solar sail design.]] | ||
The sail would be supported by centrifugal forces and connected to the payload by a complex fractal system of cables made of a graphite/polyimide material, which are connected to ballast masses to keep the whole structure taut. | |||
The whole sail has a configuration similar to a parachute. | |||
It also incorporates springs and dampers to control the dynamic behavior of the sail, as well reels and electric motors to maneuver the sail. | |||
The sail segments would each be <math>0.575 m^2</math>, as a convenient size related to choice in the original concept of carrying a factory for these things aboard the payload bay of the Space Shuttle. | |||
The triangular sail segment is connected to three catenary tensioning members that transmit the force to the rigging using foil springs. | |||
The whole sail would be made of thousands of these things, tenselated roughly in hexagon 2.4 km across. All connected to the payload by a 3D rigging that gives the name of the design. It is quite the sight! | |||
To maneuver the sail, special sail panels can be reeled out of the plane of the sail, to tilt them and create a maneuvering force. | |||
It’s also possible to reel in and out the whole sail as it rotates to keep the sail at a constant inclination against the Sun. Kinda like a helicopter cyclic control. | |||
[insert diagram] | [insert diagram] | ||
In his work, Drexler estimates that the rigging mass would only be 50% of the sail mass for a solar sail made with 15 nm films, and just 10% of the mass for a sail with 100 nm films. | |||
It’s important to have a rigging system as lightweight as possible to maximize the performance due to the use of the super thin films. | |||
As mentioned, these films are way too fragile to reliably deploy them by unfolding or unrolling. | |||
They would just tear apart, not a pretty picture! | |||
So instead, they would have to be manufactured in space. | |||
Drexler discusses the design of such a machine, capable of manufacturing the films in orbit by coating a cool metal belt with a thin layer of sublimatable solid and then coating it with a metal film by vapor deposition. | |||
Once the film is done, tiny foil springs are attached to the edges of the panel. The assembly is slightly heated up to sublimate the solid, which vaporizes and is recovered. The panel detaches from the belt and can be moved. | Once the film is done, tiny foil springs are attached to the edges of the panel. The assembly is slightly heated up to sublimate the solid, which vaporizes and is recovered. The panel detaches from the belt and can be moved. | ||
[insert diagram] | |||
Because the structure is entirely held together by tension. Assembly something like this is easier said than done. Not only the structure needs to be under tension radially, but also axially (during flight, due to the light pressure). But during assembly, it would have to be held taut by a supporting scaffolding as big as the sail itself. Which, differently from the sail, would need compression members. | |||
The whole scaffolding rotates with the sail it assembles, and robotic cranes move over the sail, with the help of cables, installing each panel. And on the great launch day, anything in front of the sail is removed, the sail is spun up to its operational specs, the sail is exposed to light, which starts to feel the force from the light pressure and…countdown…3, 2, 1…restrains release and the sail departs! Happy sailing! | The whole scaffolding rotates with the sail it assembles, and robotic cranes move over the sail, with the help of cables, installing each panel. And on the great launch day, anything in front of the sail is removed, the sail is spun up to its operational specs, the sail is exposed to light, which starts to feel the force from the light pressure and…countdown…3, 2, 1…restrains release and the sail departs! Happy sailing! | ||
[insert diagram] | |||
The most important metric of any solar sail is its total areal density, how much mass it has per unit of area. As the forces involved from radiation pressure are typically extremely low, it’s of uttermost importance that we try to minimize it as much as possible. Areal density goes beyond the sail as well, it’s important to consider rigging systems that are as lightweight as possible, if not lighter. | ==Performance / Capabilities / Applications== | ||
The most important metric of any solar sail is its total areal density, how much mass it has per unit of area. | |||
As the forces involved from radiation pressure are typically extremely low, it’s of uttermost importance that we try to minimize it as much as possible. | |||
Areal density goes beyond just the mass of the sail as well, it’s important to consider the rigging systems that are as lightweight as possible, if not lighter. | |||
Knowing the total areal density of the sail (which includes sail, rigging and payload), the acceleration can be calculated by a simple formula, where d is the areal density of the solar sail: | |||
a = 2 * If / c * d | a = 2 * If / c * d | ||
For the type of solar sails that have been tested, using metalized polymer films, the areal density, including the rigging system | For the type of solar sails that have been tested, using metalized polymer films, the areal density, including the rigging system and payload goes from | ||
something like 1610 g/m2 for IKARUS, through 156 g/m2 for LightSail 2, to 20-30 g/m2 for concepts currently being developed. | |||
Solar sails with solid rigging beams are limited to a best value of around 5 g/m2. | |||
Such solar sail would be able to accelerate at about 1.8 mm/s2 if located as far from the Sun as Earth is. | |||
That’s like gainning the speed of a snail every 7 seconds. | |||
It's slow, but on par with current ion thruster systems. | |||
Could we try to make things better? | |||
Find ways to build a lighter sail, so we can accelerate faster? | |||
Maybe. | |||
More advanced ultra-lightweight solar sails like the Lattice Sailer concept. | |||
It is designed to be fabricated and fully assembled in space, as its structures would be far too frail to survive a launch and deployment. | |||
Theoretically, such solar sail could reach densities as low as 70 mg/m2. | |||
With these advanced sails, spacecraft could accelerate at over 0.1 m/s2 under the same conditions, which is pretty good! | |||
It’s important to note, that the thrust is proportional to the incident irradiance, the amount of light the sail receives. And that falls with the square of the distance, the good ol’ inverse square law. As a result, the thrust generated by a solar sail drops with the square of the distance it is from the star. A solar Sail near Jupiter would produce over 25 times less thrust than near Earth, because Jupiter is over 5 times farther from the Sun than the Earth. | It’s important to note, that the thrust is proportional to the incident irradiance, the amount of light the sail receives. | ||
And that falls with the square of the distance, the good ol’ inverse square law (always there to make our job harder). | |||
As a result, the thrust generated by a solar sail drops with the square of the distance it is from the star. | |||
A solar Sail near Jupiter would produce over 25 times less thrust than near Earth, because Jupiter is over 5 times farther from the Sun than the Earth. | |||
[insert diagram] | [insert diagram] | ||
Because solar sails use the very weak radiation pressure from light, and are built very frail, they can only work in space. And around planets with atmospheres, | Because solar sails use the very weak radiation pressure from light, and are built very frail, they can only work in space. | ||
And around planets with atmospheres, we have to be careful to only operate them above a given obital altitude. | |||
Try to go below that, and the atmospheric drag (even at orbital altitudes), would overpower the trust from the sail. | |||
We would have a bad airbrake instead of a solar sail, not good at all! | |||
On Earth, that’s about 800 km, but under times of low solar activity, that can go as low as 500 km, so watch out! | |||
Solar sails always create a thrust force that points normal to the plane of the sail. But at the same time, they need to show the reflective surface to the Sun, this limits the kind of maneuvers solar sails can do. | Solar sails always create a thrust force that points normal to the plane of the sail. | ||
But at the same time, they need to show the reflective surface to the Sun, this limits the kind of maneuvers solar sails can do. | |||
If a sail can produce a force that is higher than the local force of gravity (usually only the high performing sails can do that) is also something to consider. | |||
If the sail is aligned with the sun, so the thrust force points radially out, but is not powerful enough to accelerate away from the sun. | |||
It will apply a radial force that will counterbalance the gravitational force, and increase the orbital altitude, one quarter of orbit ahead. | |||
And also reduce orbital altitude one quarter of orbit behind (just like in KSP). | |||
If the | If you do that throughout the whole orbit, it will be as if gravity was weaker, and your orbit will be displaced outward. | ||
So you can keep orbiting at a given altitude at slower speed than normal. | |||
A slight variation of this effect is to angle the sail a bit above or below the orbital plane, so you are pushed not just outward. | |||
But also above or below the orbital plane. | |||
This creates a displaced orbit that is not possible with gravity alone (non-Keplerian orbit). | |||
[[Image:Displaced_orbit.png|thumb|left|Out of plane orbit.]] | |||
One alternative is to use the sail during only one segment of the orbit, which will allow the user to control the lowest and highest points of the orbit, still not very useful. | |||
For weak sails, what you really want to do is to point the sail at an angle, towards the orbital motion. | |||
The force, generated in the same direction will have two components, one radial, that over a whole orbit gets canceled as before. | |||
But we also get one component that is aligned with the orbital motion, this will keep increasing the speed of the solar sail in orbit, causing it to spiral out. | |||
Yey, we can now go to places! | |||
If the sail is made to point in an angle to the other side, we can make the sail lose orbital speed and spiral inwards. | |||
And that’s how we tack a solar sail…Yarr, Matey! | |||
[insert diagram of tacking]spiral_trajectory.png | |||
[[Image:spiral_trajectory.png|thumb|left|Solar sail spiralling outwards away from the Sun.]] | |||
[[Image:spiral_trajectory_in.png|thumb|right|Solar sail moving closer to Sun.]] | |||
We can also use a large body to our advantage. | |||
A solar sail around Earth for instance, could point pretty much straight at the Sun to accelerate, during half the orbit, and then point sideways to the Sun during the other half. | |||
We can also use a large body to our advantage. A solar sail around Earth for instance, could point pretty much straight at the Sun to accelerate, during half the orbit, and then point sideways to the Sun during the other half. So it can quickly rise or lower its orbit. | So it can quickly rise or lower its orbit. | ||
[insert diagram] | [insert diagram] | ||
Solar sails could also be used to produce small forces to keep a satellite in a fixed orbit or in a specific position in relation to another spacecraft or body, station-keeping | Solar sails could also be used to produce small forces to keep a satellite in a fixed orbit or in a specific position in relation to another spacecraft or body, station-keeping. | ||
But without having to use any propellant. | |||
For many satellites and space probes, the internal reserve of fuel for maneuvering limits how long their missions can be. | |||
Back to using the sail in radial thrust mode. It might not be very useful to take you anywhere…it does allow you to change where you are though. An orbit is a | Back to using the sail in radial thrust mode. | ||
It might not be very useful to take you anywhere…it does allow you to change where you are though. | |||
An orbit is a well balanced equilibrium between the gravitational forces and inertia of a moving object. | |||
The object doesn’t fall into the central object, or flies away. | |||
A solar sail in orbit around a star can use light pressure to counterbalance the gravity of the star (even if partially), to achieve new trajectories that gravity alone wouldn’t allow. | |||
For instance, a solar sail can orbit a star at a given point, at a speed lower than the orbital speed required at that point for a normal orbit. | |||
[insert diagram] | [insert diagram] | ||
The extreme case of that situation is when the radiation pressure on the sail can fully balance the gravitational forces. Allowing an object to just float above the star, a static satellite, or statite. Large solar sails could also hover above the poles of a planet to bath them in sunlight, while using radiation pressure to balance gravity. | The extreme case of that situation is when the radiation pressure on the sail can fully balance the gravitational forces. | ||
Allowing an object to just float above the star, a static satellite, or statite. | |||
Large solar sails could also hover above the poles of a planet to bath them in sunlight, while using radiation pressure to balance gravity. | |||
[Diagram] | [Diagram] | ||
A fun kirk of a statite, is that, gravitational forces roughly fall with the square of the distance | A fun kirk of a statite, is that, gravitational forces roughly fall with the square of the distance. | ||
But radiation pressure also falls with the square of the distance, because that’s what sunlight does (good ol’ inverse square law). | |||
As result, a statite can remain in equilibrium anywhere around the star, and move anywhere (although sometimes slowly) by just adjusting the balance and the direction in which the sail points. | |||
One way to enhance the operational capabilities of a sail is to send more light to it, or send light from a different direction. In the form of a laser beam. Allowing us to illuminate a solar sail with more light that it could gather naturally from the sun, and accelerate faster. A laser beam could compensate for the loss of thrust a solar sails gets as it goes away from the Sun. Or even, slow down an incoming solar sail at a given location. | One way to enhance the operational capabilities of a sail is to send more light to it, or send light from a different direction. | ||
In the form of a laser beam. Allowing us to illuminate a solar sail with more light that it could gather naturally from the sun, and accelerate faster. | |||
A laser beam could compensate for the loss of thrust a solar sails gets as it goes away from the Sun. | |||
Or even, slow down an incoming solar sail at a given location. | |||
The problem is, just as with the photon drive, this is insanely energy intensive. | |||
[insert image] | [insert image] | ||
Also because thrust increases as one gets closer to the Sun. One maneuver proposed is to put a solar sail in a trajectory that takes it really rally close to the Sun. Like, imagine how close the Parker Solar Probe is planning to get to the Sun, and now you think even closer (Toasty!). The idea is to use the sail to produce a lot of thrust, during a relatively short period of time as the sail passes the closes to the star. This big impulse could send a solar sail to | Also because thrust increases as one gets closer to the Sun. | ||
One maneuver proposed is to put a solar sail in a trajectory that takes it really rally close to the Sun. | |||
Like, imagine how close the Parker Solar Probe is planning to get to the Sun, and now you think even closer (Toasty!). | |||
The idea is to use the sail to produce a lot of thrust, during a relatively short period of time as the sail passes the closes to the star. | |||
This big impulse could send a solar sail to distant places, or maybe even interstellar (although not that fast)! | |||
Some call that maneuver a Sun dive. | |||
[insert image] | [insert image] | ||
Line 187: | Line 305: | ||
Maybe one of the first to propose the idea of sailing in space was Johannes Kepler (1571-1630) who wrote to Galileo in 1610, "Provide ships or sails adapted to the heavenly breezes, and there will be some who will brave even that void." after noting how comet tails always point away from the Sun, as if being blown away by some sort of “solar wind”. So he imagined ships that could harness these winds to venture the cosmos. | Maybe one of the first to propose the idea of sailing in space was Johannes Kepler (1571-1630) who wrote to Galileo in 1610, "Provide ships or sails adapted to the heavenly breezes, and there will be some who will brave even that void." after noting how comet tails always point away from the Sun, as if being blown away by some sort of “solar wind”. So he imagined ships that could harness these winds to venture the cosmos. | ||
Much later after that, James Clerk Maxwell (1831-1879), with his unified theory of electromagnetism, shows that light has momentum and thus can exert pressure on objects. That’s the fundamental idea that allows light to be used as a form of propulsion. Later on, Pyotr Nikolaevich Lebedev (1866-1912), using an extremely sensitive torsional balance, demonstrated experimentally that light indeed can exert pressure on objects. | Much later after that, James Clerk Maxwell (1831-1879), with his unified theory of electromagnetism, shows that light has momentum and thus can exert pressure on objects. | ||
That’s the fundamental idea that allows light to be used as a form of propulsion. | |||
Later on, Pyotr Nikolaevich Lebedev (1866-1912), using an extremely sensitive torsional balance, demonstrated experimentally that light indeed can exert pressure on objects. | |||
Even Konstantin Tsiolkovsky (1857-1935), the father of spaceflight, has proposed using sunlight radiation pressure to travel through space, suggesting "using tremendous mirrors of very thin sheets to utilize the pressure of sunlight to attain cosmic velocities". | Even Konstantin Tsiolkovsky (1857-1935), the father of spaceflight, has proposed using sunlight radiation pressure to travel through space, suggesting "using tremendous mirrors of very thin sheets to utilize the pressure of sunlight to attain cosmic velocities". | ||
Friedrich Zander (1887-1933) published a technical paper in 1925 laying down the first technical analysis of solar sailing. But many came to hear about the concept through Carl Sagan (1934-1996), who popularized the concept of solar sailing through his lectures, books and television shows. Being specially interested in the idea of one such spacecraft to perform a rendezvous with Halley’s comet. An idea the JPL studied seriously in 1976. | Friedrich Zander (1887-1933) published a technical paper in 1925 laying down the first technical analysis of solar sailing. But many came to hear about the concept through Carl Sagan (1934-1996), who popularized the concept of solar sailing through his lectures, books and television shows. | ||
On June 21, 2005, a joint private project between the Planetary Society, Cosmos Studios and the Russian Academy of Science launched a prototype solar sail called Cosmos 1 in a Volna rocket launched from a submarine | Being specially interested in the idea of one such spacecraft to perform a rendezvous with Halley’s comet. | ||
On November 19, 2010, NASA launched NanoSail-D2 with FASTSAT aboard a Minotaur IV, becoming NASA’s first solar sail deployed in low earth orbit. NanoSail-D1 was lost on a Falcon 1 failed launch. | An idea the JPL studied seriously in 1976. | ||
On 20 May 2015, the Planetary Society launched a second solar sail called “LightSail-1” to demonstrate satellite systems for the following LightSail-2. Deployment happened in low earth orbit. Following up, on 25 June 2019, LightSail-2 was launched as a secondary payload from a Falcon Heavy launch, the sail was deployed at a much higher orbit and was able to demonstrate orbital maneuvering using the sail. | |||
On June 21, 2005, a joint private project between the Planetary Society, Cosmos Studios and the Russian Academy of Science launched a prototype solar sail called Cosmos 1 in a Volna rocket launched from a submarine. | |||
But the rocket failed and the spacecraft never reached orbit. | |||
Five years later, on 21 May 2010, JAXA launched the world’s first interplanetary solar sail IKAROS to Venus to demonstrate solar sail flight, together with the AKATSUKI probe. | |||
On November 19, 2010, NASA launched NanoSail-D2 with FASTSAT aboard a Minotaur IV, becoming NASA’s first solar sail deployed in low earth orbit. | |||
NanoSail-D1 was lost on a Falcon 1 failed launch. | |||
On 20 May 2015, the Planetary Society launched a second solar sail called “LightSail-1” to demonstrate satellite systems for the following LightSail-2. | |||
Deployment happened in low earth orbit. Following up, on 25 June 2019, LightSail-2 was launched as a secondary payload from a Falcon Heavy launch, the sail was deployed at a much higher orbit and was able to demonstrate orbital maneuvering using the sail. | |||
==Worked Example(s)== | ==Worked Example(s)== | ||
To date, only a | To date, only a handful of solar sails have actually been worked on, built and actually flown with the intention of testing solar sail propulsion. | ||
Here are a few. | |||
===Cosmos 1=== | ===Cosmos 1=== | ||
Cosmos 1 was a joint project between Cosmos Studios and The Planetary Society, to test a solar sail in space. An uncrewed spacecraft was launched on 21 | Cosmos 1 was a joint project between Cosmos Studios and The Planetary Society, to test a solar sail in space. | ||
An uncrewed spacecraft was launched on June 21 2005 aboard a small Volna rocket launched from a Russian submarine. But the launch failed to reach orbit. | |||
The spacecraft itself weighed around 100 kg and consisted of eight triangular blades connected to a central hub. | |||
The Rigging system consisted of inflatable structural tubes. Total surface area of the sail was about <math> 600 m^2 </math> and was made of PET film coated in aluminum. | |||
The mission was expected to last 30 days, during which the spacecraft would use its sails to boost its orbit by up to 100 km. | |||
Had it gone as planned, Cosmos 1 would be the first spacecraft to use a solar sail as propulsion. | |||
[ | [[Image:Cosmos1_in_orbit.jpg|thumb|left|Artist's concept of Cosmos 1.]] | ||
The spacecraft | ===LightSail 1 and 2=== | ||
The LightSail project was a follow up on the failed Cosmos 1 by The Planetary Society. | |||
Two spacecraft were launched, LightSail 1 as an engineering demonstration to test the sail deployment system in orbit. | |||
And LightSail 2, to demonstrate using solar sails as propulsion. | |||
Each spacecraft was built around a 3U cubesat bus, about 30cm x 10 cm x 10 cm in their stowed configuration. | |||
And capable of deploying a Mylar solar sail with an area of 32 m2. The sail was rigged with four 4-meter long booms made of lightweight cobalt alloy. | |||
[ | [[Image:LightSail1.jpg|thumb|left|LightSail 1 deploying its sail on 8 June 2015.]] | ||
LightSail 1 was launched on 20 May 2015 as a secondary payload on a Atlas V launch. | |||
Successfully deploying its sail on 7 June 2015, and reentering the atmosphere one week later. | |||
LightSail 2 was launched as a secondary payload on a Falcon Heavy launch on 25 June 2019. | |||
Deployed its sail almost a month later on 23 July 2019. | |||
And it seems to have demonstrated some limited maneuvering using the solar sail. | |||
[[Image:LightSail_2.png|thumb|right|LightSail 2 deploying its sail on 23 July 2019.]] | |||
===IKARUS=== | ===IKARUS=== | ||
IKAROS (Interplanetary Kite-craft Accelerated by Radiation Of the Sun) is an experimental spacecraft built by JAXA and launched on 20 May 2020, aboard a H-IIA rocket together with the Akatsuki mission, and four other small spacecraft. IKARUS was the first spacecraft to demonstrate solar sail propulsion in interplanetary space. It flew just just over 80 thousand kilometers from Venus. And by 2013, it had changed the spacecraft’s speed by over 400 meters per second. | |||
[ | IKAROS (Interplanetary Kite-craft Accelerated by Radiation Of the Sun) is an experimental spacecraft built by JAXA and launched on 20 May 2020, aboard a H-IIA rocket together with the Akatsuki mission, and four other small spacecraft. | ||
IKARUS was the first spacecraft to demonstrate solar sail propulsion in interplanetary space. | |||
[ | It flew just just over 80 thousand kilometers from Venus. | ||
And by 2013, it had changed the spacecraft’s speed by over 400 meters per second. | |||
[[Image:IKAROS.jpg|thumb|left|Artist's concept of IKAROS in flight.]] | |||
IKAROS sail was made of metalized polyimide film, with integrated LCD panels to adjust reflectance for attitude control. | |||
It also had thin film solar cells built into the sail. | |||
The sail was deployed by spinning the spacecraft and releasing four 500 gram tip masses connected to lines on the diagonals of the sail, which then stretched the sail by centrifugal force. | |||
[[Image:IKAROS2.jpg|thumb|right|Photo showing IKAROS' solar sails deployed on 14 June, 2010.]] | |||
===NanoSail-D and D2=== | ===NanoSail-D and D2=== | ||
NanoSail-D was a small spacecraft built by NASA's Ames Research Center to study solar sail deployment in space. Built around a 3U CubeSat and weighing about 4 kg. It launched aboard a Falcon 1 rocket on 3 August 2008. But the launch failed, and the payload never reached orbit. | |||
NanoSail-D2 was originally built as the ground spare for NanoSail-D. After the failed launch in 2008, the spare received several improvements and launched on a Minotaur IV rocket on 20 November 2010. The spacecraft was deployed on 19 January 2011, after the ejection mechanism failed, but ultimately released the spacecraft. | NanoSail-D was a small spacecraft built by NASA's Ames Research Center to study solar sail deployment in space. | ||
Built around a 3U CubeSat and weighing about 4 kg. | |||
It launched aboard a Falcon 1 rocket on 3 August 2008. | |||
But the launch failed, and the payload never reached orbit. | |||
NanoSail-D2 was originally built as the ground spare for NanoSail-D. | |||
After the failed launch in 2008, the spare received several improvements and launched on a Minotaur IV rocket on 20 November 2010. | |||
The spacecraft was deployed on 19 January 2011, after the ejection mechanism failed, but ultimately released the spacecraft. | |||
[[Image:NanoSail-D.jpg|thumb|right|Artist's concept of NanoSail-D in orbit.]] | |||
==Additional Reading== | ==Additional Reading== | ||
[ | [https://www.galacticlibrary.net/wiki/Electric_Sail Electric Sail] | ||
[ | [https://www.galacticlibrary.net/wiki/Magnetic_Sail Magnetic Sail] | ||
[https://www.galacticlibrary.net/wiki/Laser_Propulsion Laser Sail] | |||
===Additional References=== | ===Additional References=== | ||
<references group="footnotes"/> | |||
Solar Sails: A Novel Approach to Interplanetary travel - Giovanni Vulpetti Les Johnson Gregory L. Matloff | Solar Sails: A Novel Approach to Interplanetary travel - Giovanni Vulpetti Les Johnson Gregory L. Matloff | ||
Solar Sailing: Technology, Dynamis and Mission Applications - Colin R. Mclnnes | Solar Sailing: Technology, Dynamis and Mission Applications - Colin R. Mclnnes | ||
- Kim Eric Drexler | |||
The Heliogyro, an interplanetary flying machine | The Heliogyro, an interplanetary flying machine | ||
<ref name="lattice_sailer">K. Eric Drexler, ''Design of a High Performance Solar Sail System'', (New York: Massachusetts Institute of Technology, 1979).</ref> | |||
[[Category:Transportation & Infrastructure]][[Category:Transportation]][[Category:Propulsion]][[Category:Propulsion - Harvested Energy, Harvested Reaction Mass]] |
Latest revision as of 14:51, 23 April 2024
Solar sails, or more specifically solar photon sails, use the radiation pressure from the light emitted from the Sun to propel spacecraft through space without using any propellant. In an almost poetically similar manner to a sail sea ship. Light is reflected from huge, extremely thin, reflective sheets made as light as possible, sometimes too light to survive any force much larger than the radiation pressure from the light. They can only be used in space. The “sails” are extended over some “rigging” structure that keeps the sail taut and attaches the payload to the solar sail so it can be carried away.
Solar sails can be used for many different purposes, typically space maneuvers that require little thrust and are not significantly limited in duration. They can be used for station keeping, orbital maneuvering or interplanetary propulsion. They typically accelerate very slowly, like ion-engines. Some more optimized designs can pick up tremendous speeds given enough time and adequate materials and construction.
Introduction
Solar sails, also known as photon sails or light sails, are devices that can exploit an interesting quirk of relativity for propulsion. When a photon is absorbed on or reflected from a surface, it applies a force on it. This is denominated radiation pressure, and is typically a very tiny tiny value (did I say tiny?). So, if we deploy a very large reflective surface to reflect photons, that can also be made to be extremely thin and lightweight, it’s possible to produce a substantial force to push a spacecraft around so you can go to places, not unlike a sailing sea vessel. With the great advantage of not consuming any propellant while doing that, so you don’t have to carry any! This makes solar sails a propellantless propulsion system, and so we don’t have to worry about that pesky rocket equation.
Somebody once said there is no free lunch, and with solar sails we can have a propellant-free propulsion, but typically solar sails don’t generate much force, putting them in the same category of low-thrust methods like ion-engines. Due to their tiny thrust, the sails must operate in space, handling inter-orbit transits and interplanetary trajectories. Most solar sails are too heavy to accelerate faster than the local gravity, and thus need to spiral in or out of gravity wells like low power electric thrusters. Although a really lightweight high performance solar sail could perform direct injections into interplanetary space relatively quickly. And even float over a star or a planet, as in a statite. Allowing us to build all sorts of crazy systems and structures.
Propulsion Performance
Performance of solar sails vary wildly among the several designs that have been proposed. The design performance presented here comes from the concept proposed by Kim Eric Drexler (yes, the nanotech guy) in his 1977 Master’s Thesis. It’s a very high performance design that has inspired the photon sail design seen in “Rocheworld” by Robert L. Forward. Figures here do not assume a payload, so the sail can be used as an Engine, like an ion thruster.
Name | Lattice Sailer |
Thrust | 7.26 N |
Mass | 100 kg |
Efficiency | 80% |
Power Consumption | 1.36 GW |
T/W | 0.0074 (Calculated) |
Energy Source (Fuel) | Sun |
Propellant | Solar Photons |
Specific Power | 13.6 MW/kg (Calculated) |
Conditions are also taken as about 1 AU from the Sun. Assuming a sail capable of reflecting 80% of the incoming light, with an areal density , an area of and pointed directly at the Sun.
Design and Function
As described by relativity, photons of light might not have mass in a classical sense, but they carry momentum. So when a photon hits a surface, and sometimes bounces off it (emitting photons also works, that’s how the photon drive works), we have an exchange of momentum, not unlike a ball hitting a wall. The sum of all the tiny, tiny exchanges of momentum with a ridiculously large number of photons exerts a pressure on the surface.
[inset diagram]
We call that radiation pressure, and under normal conditions, it’s usually very weak. But nonetheless, this pressure created by light, applied over a large surface creates a force that can move a spacecraft. For a surface that is perfectly absorbing, the radiation pressure can be calculated by dividing the incident irradiance (usually measured in ), by the speed of light c (in ):
When the surface reflects a photon, it gets an exchange of momentum when the photon comes, and also when it is reflected back. So we get twice the pressure!
Using this formula, we can calculate that the radiation pressure around Earth’s orbit from sunlight, where the incident irradiance is about is: [solar constant value]
So the pressure is just a bit over 9 micropascals, that’s about the pressure due to the weight of a small ant, distributed over one entire square meter! It’s nuts! But in space, we don’t have air resistance, so even a small force, applied continuously over a long period of time can really add up. Even more so, if we use extremely large and thin sails, that are super lightweight! They don’t need to be very strong, as they don’t have to support their own weight in space. And can accumulate a large enough force to allow for reasonable fast accelerations.
Light sails can have many configurations. But basically they contain three components. The payload, which is usually the spacecraft you want to move around. Attached to the payload is the sail and a system of booms and rigging to keep the sail taut and keep the sail in the right position and orientation.
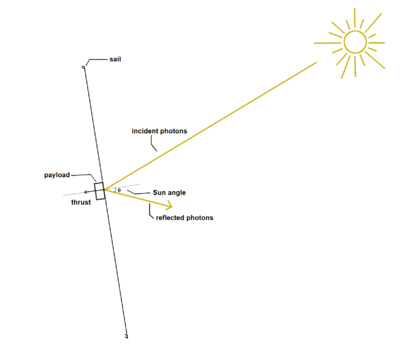
Because radiation pressure is so weak, we want to build the most lightweight sail possible so we can generate as much force as we can, without also getting too much extra mass to accelerate. So solar sails are made of extremely thin reflective films of a wide range of materials. From films of polymers like polyimide or polyesters covered in an extremely thin metal layer applied by vapor deposition (like those space blankets), to extremely thin films of metal that are just nanometers thick, to more exotic materials like extremely thin layered dielectrics materials. Even graphene has been proposed (and tested in the lab) as solar sail material.
But also, as important as the sail, the rigging should not be neglected. Several types of rigging have been proposed for solar sails, from extremely lightweight trusses and inflatable booms that support and keep the sail stretched, to designs that are supported by centrifugal forces created by spinning the whole solar sail, and having masses at the edge to keep the whole thing taut. To very speculative designs that bounce some of the incoming light inside the structure of the sail to keep it inflated.
Sail Configurations
Heliogyro
The Heliogyro is a 1967 concept for a new type of solar sail proposed by Richard H. MacNeal from the Astro Research Corporation. The vehicle employs long, narrow blades constructed from thin films that are rotated around the spacecraft bus in a manner similar to a helicopter rotor. Another important feature is the deployment process, simply done by unrolling the sail blades from spools.
To keep the sail stretched and in a given attitude, the blades are spun around to create centrifugal force to maintain them under tension. In one of the 1967 designs, one concept of the Heliogyro uses two blades, which are narrow sheets of Mylar with a thin film of aluminum, to make them reflective. They are around meters wide and km long, very narrow and super long, with a thickness of about micrometers. To stop the blades from curling over the width, the blades feature a small compression batten every 30.5 meters or so.

For maneuvering, a solar sail must be able to control how much sunlight it is reflecting, to control the thrust, but also be able to turn around and change its attitude. Especially if it is tacking. In the heliogyro, the blades are attached to the main spacecraft by spools, which can rotate around the blade's longest axis. If the heliogyro is pointing at the Sun, this allows the control of how much sunlight is being reflected. This works similarly to the collective control of a helicopter. To cause the spacecraft to turn around, the pitch of the blades is changed as the blade rotates around. In a similar way to cyclic pitch control, also from helicopters. That way, it is possible to apply force on just one side of the sail to make it turn around. Both controls can be combined to do all sorts of maneuvers.
There have been few studies on heliogyros, but one of the most detailed was done in 1977 by the JPL for a mission for a rendezvous with Comet Halley. That design was even larger, with blades meters wide and km long. Although in the end they preferred a solar electric propulsion concept over an untested solar sail technology like the Heliogyro.
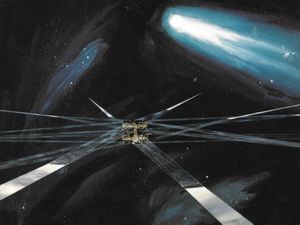
More recently, there was a 1989 study by an MIT team for a mission to Mars. With a smaller Heliogyro, using 8 blades, 100 meters wide. More recently, in 2014, NASAA performed a study on a possible Heliogyro demonstration mission called HELIOS (High-Performance, Enabling, Low-Cost, Innovative, Operational Solar Sail). The demonstrator would use six blades that would be 4.5 meters wide and 220 meters long.
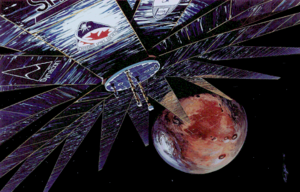
Because most Heliogyro concepts feature vehicles that are launched from Earth and then have to deploy their sails, they usually feature relatively "thick" Mylar sheets. With that kind of limitation, most Heliogyro are usually limited to accelerations of the order of when near Earth. It's no neck breaking acceleration, but in space, even that can build up. Just to get an idea, you can gain around one kilometer per second of delta-V in just 8 days with that.
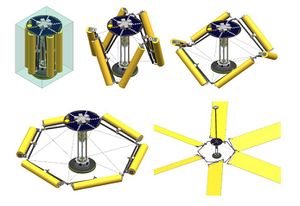
The Lattice Sailer
In 1979, Kim Eric Drexler (the big guy behind nanotechnology) received his M.S. with a Master’s thesis titled “Design of a High Performance Solar Sail System”, where he described the design of a very high performance solar sail based on ultrathin aluminum films and a centrifugally stabilized structure, that he called the “Lattice Sailer” due to its rigging system. Instead of using thin plastic films covered with reflective metallic coats, the Lattice sailer makes use of thin aluminum films, in the range of tens of nanometers thick, that would be manufactured in space (such films are way too delicate to survive a launch and a deployment). The sail would be built of many smaller triangular segments. Each with a thickness ranging from 15 to 100 nm, and would only weigh , tens of times lighter than the metallized plastic films. The sheets of this metallic film are covered in a pattern of small cuts and wrinkles that can remove tension in small areas of the film to stop tears from propagating.
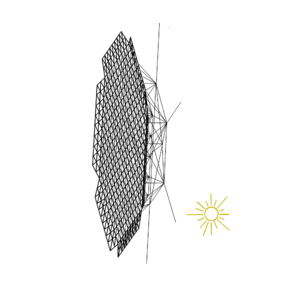
The sail would be supported by centrifugal forces and connected to the payload by a complex fractal system of cables made of a graphite/polyimide material, which are connected to ballast masses to keep the whole structure taut. The whole sail has a configuration similar to a parachute. It also incorporates springs and dampers to control the dynamic behavior of the sail, as well reels and electric motors to maneuver the sail. The sail segments would each be , as a convenient size related to choice in the original concept of carrying a factory for these things aboard the payload bay of the Space Shuttle. The triangular sail segment is connected to three catenary tensioning members that transmit the force to the rigging using foil springs.
The whole sail would be made of thousands of these things, tenselated roughly in hexagon 2.4 km across. All connected to the payload by a 3D rigging that gives the name of the design. It is quite the sight! To maneuver the sail, special sail panels can be reeled out of the plane of the sail, to tilt them and create a maneuvering force. It’s also possible to reel in and out the whole sail as it rotates to keep the sail at a constant inclination against the Sun. Kinda like a helicopter cyclic control.
[insert diagram]
In his work, Drexler estimates that the rigging mass would only be 50% of the sail mass for a solar sail made with 15 nm films, and just 10% of the mass for a sail with 100 nm films.
It’s important to have a rigging system as lightweight as possible to maximize the performance due to the use of the super thin films.
As mentioned, these films are way too fragile to reliably deploy them by unfolding or unrolling.
They would just tear apart, not a pretty picture!
So instead, they would have to be manufactured in space.
Drexler discusses the design of such a machine, capable of manufacturing the films in orbit by coating a cool metal belt with a thin layer of sublimatable solid and then coating it with a metal film by vapor deposition.
Once the film is done, tiny foil springs are attached to the edges of the panel. The assembly is slightly heated up to sublimate the solid, which vaporizes and is recovered. The panel detaches from the belt and can be moved.
[insert diagram]
Because the structure is entirely held together by tension. Assembly something like this is easier said than done. Not only the structure needs to be under tension radially, but also axially (during flight, due to the light pressure). But during assembly, it would have to be held taut by a supporting scaffolding as big as the sail itself. Which, differently from the sail, would need compression members.
The whole scaffolding rotates with the sail it assembles, and robotic cranes move over the sail, with the help of cables, installing each panel. And on the great launch day, anything in front of the sail is removed, the sail is spun up to its operational specs, the sail is exposed to light, which starts to feel the force from the light pressure and…countdown…3, 2, 1…restrains release and the sail departs! Happy sailing!
[insert diagram]
Performance / Capabilities / Applications
The most important metric of any solar sail is its total areal density, how much mass it has per unit of area. As the forces involved from radiation pressure are typically extremely low, it’s of uttermost importance that we try to minimize it as much as possible. Areal density goes beyond just the mass of the sail as well, it’s important to consider the rigging systems that are as lightweight as possible, if not lighter. Knowing the total areal density of the sail (which includes sail, rigging and payload), the acceleration can be calculated by a simple formula, where d is the areal density of the solar sail:
a = 2 * If / c * d
For the type of solar sails that have been tested, using metalized polymer films, the areal density, including the rigging system and payload goes from something like 1610 g/m2 for IKARUS, through 156 g/m2 for LightSail 2, to 20-30 g/m2 for concepts currently being developed. Solar sails with solid rigging beams are limited to a best value of around 5 g/m2. Such solar sail would be able to accelerate at about 1.8 mm/s2 if located as far from the Sun as Earth is. That’s like gainning the speed of a snail every 7 seconds. It's slow, but on par with current ion thruster systems.
Could we try to make things better? Find ways to build a lighter sail, so we can accelerate faster? Maybe. More advanced ultra-lightweight solar sails like the Lattice Sailer concept. It is designed to be fabricated and fully assembled in space, as its structures would be far too frail to survive a launch and deployment. Theoretically, such solar sail could reach densities as low as 70 mg/m2. With these advanced sails, spacecraft could accelerate at over 0.1 m/s2 under the same conditions, which is pretty good!
It’s important to note, that the thrust is proportional to the incident irradiance, the amount of light the sail receives. And that falls with the square of the distance, the good ol’ inverse square law (always there to make our job harder). As a result, the thrust generated by a solar sail drops with the square of the distance it is from the star. A solar Sail near Jupiter would produce over 25 times less thrust than near Earth, because Jupiter is over 5 times farther from the Sun than the Earth.
[insert diagram]
Because solar sails use the very weak radiation pressure from light, and are built very frail, they can only work in space. And around planets with atmospheres, we have to be careful to only operate them above a given obital altitude. Try to go below that, and the atmospheric drag (even at orbital altitudes), would overpower the trust from the sail. We would have a bad airbrake instead of a solar sail, not good at all! On Earth, that’s about 800 km, but under times of low solar activity, that can go as low as 500 km, so watch out!
Solar sails always create a thrust force that points normal to the plane of the sail. But at the same time, they need to show the reflective surface to the Sun, this limits the kind of maneuvers solar sails can do. If a sail can produce a force that is higher than the local force of gravity (usually only the high performing sails can do that) is also something to consider. If the sail is aligned with the sun, so the thrust force points radially out, but is not powerful enough to accelerate away from the sun. It will apply a radial force that will counterbalance the gravitational force, and increase the orbital altitude, one quarter of orbit ahead. And also reduce orbital altitude one quarter of orbit behind (just like in KSP).
If you do that throughout the whole orbit, it will be as if gravity was weaker, and your orbit will be displaced outward. So you can keep orbiting at a given altitude at slower speed than normal. A slight variation of this effect is to angle the sail a bit above or below the orbital plane, so you are pushed not just outward. But also above or below the orbital plane. This creates a displaced orbit that is not possible with gravity alone (non-Keplerian orbit).
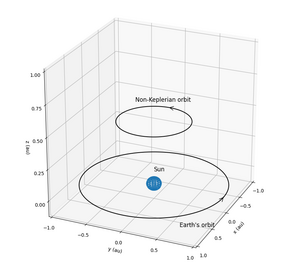
One alternative is to use the sail during only one segment of the orbit, which will allow the user to control the lowest and highest points of the orbit, still not very useful. For weak sails, what you really want to do is to point the sail at an angle, towards the orbital motion. The force, generated in the same direction will have two components, one radial, that over a whole orbit gets canceled as before. But we also get one component that is aligned with the orbital motion, this will keep increasing the speed of the solar sail in orbit, causing it to spiral out. Yey, we can now go to places! If the sail is made to point in an angle to the other side, we can make the sail lose orbital speed and spiral inwards. And that’s how we tack a solar sail…Yarr, Matey!
[insert diagram of tacking]spiral_trajectory.png

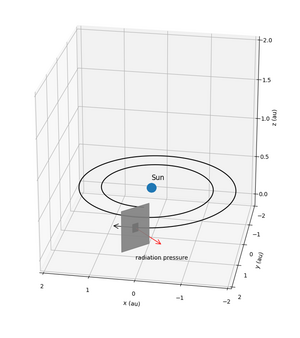
We can also use a large body to our advantage. A solar sail around Earth for instance, could point pretty much straight at the Sun to accelerate, during half the orbit, and then point sideways to the Sun during the other half. So it can quickly rise or lower its orbit.
[insert diagram]
Solar sails could also be used to produce small forces to keep a satellite in a fixed orbit or in a specific position in relation to another spacecraft or body, station-keeping. But without having to use any propellant. For many satellites and space probes, the internal reserve of fuel for maneuvering limits how long their missions can be.
Back to using the sail in radial thrust mode. It might not be very useful to take you anywhere…it does allow you to change where you are though. An orbit is a well balanced equilibrium between the gravitational forces and inertia of a moving object. The object doesn’t fall into the central object, or flies away. A solar sail in orbit around a star can use light pressure to counterbalance the gravity of the star (even if partially), to achieve new trajectories that gravity alone wouldn’t allow. For instance, a solar sail can orbit a star at a given point, at a speed lower than the orbital speed required at that point for a normal orbit.
[insert diagram]
The extreme case of that situation is when the radiation pressure on the sail can fully balance the gravitational forces. Allowing an object to just float above the star, a static satellite, or statite. Large solar sails could also hover above the poles of a planet to bath them in sunlight, while using radiation pressure to balance gravity.
[Diagram]
A fun kirk of a statite, is that, gravitational forces roughly fall with the square of the distance. But radiation pressure also falls with the square of the distance, because that’s what sunlight does (good ol’ inverse square law). As result, a statite can remain in equilibrium anywhere around the star, and move anywhere (although sometimes slowly) by just adjusting the balance and the direction in which the sail points.
One way to enhance the operational capabilities of a sail is to send more light to it, or send light from a different direction. In the form of a laser beam. Allowing us to illuminate a solar sail with more light that it could gather naturally from the sun, and accelerate faster. A laser beam could compensate for the loss of thrust a solar sails gets as it goes away from the Sun. Or even, slow down an incoming solar sail at a given location. The problem is, just as with the photon drive, this is insanely energy intensive.
[insert image]
Also because thrust increases as one gets closer to the Sun. One maneuver proposed is to put a solar sail in a trajectory that takes it really rally close to the Sun. Like, imagine how close the Parker Solar Probe is planning to get to the Sun, and now you think even closer (Toasty!). The idea is to use the sail to produce a lot of thrust, during a relatively short period of time as the sail passes the closes to the star. This big impulse could send a solar sail to distant places, or maybe even interstellar (although not that fast)! Some call that maneuver a Sun dive.
[insert image]
History / Development
Maybe one of the first to propose the idea of sailing in space was Johannes Kepler (1571-1630) who wrote to Galileo in 1610, "Provide ships or sails adapted to the heavenly breezes, and there will be some who will brave even that void." after noting how comet tails always point away from the Sun, as if being blown away by some sort of “solar wind”. So he imagined ships that could harness these winds to venture the cosmos. Much later after that, James Clerk Maxwell (1831-1879), with his unified theory of electromagnetism, shows that light has momentum and thus can exert pressure on objects.
That’s the fundamental idea that allows light to be used as a form of propulsion. Later on, Pyotr Nikolaevich Lebedev (1866-1912), using an extremely sensitive torsional balance, demonstrated experimentally that light indeed can exert pressure on objects. Even Konstantin Tsiolkovsky (1857-1935), the father of spaceflight, has proposed using sunlight radiation pressure to travel through space, suggesting "using tremendous mirrors of very thin sheets to utilize the pressure of sunlight to attain cosmic velocities".
Friedrich Zander (1887-1933) published a technical paper in 1925 laying down the first technical analysis of solar sailing. But many came to hear about the concept through Carl Sagan (1934-1996), who popularized the concept of solar sailing through his lectures, books and television shows. Being specially interested in the idea of one such spacecraft to perform a rendezvous with Halley’s comet. An idea the JPL studied seriously in 1976.
On June 21, 2005, a joint private project between the Planetary Society, Cosmos Studios and the Russian Academy of Science launched a prototype solar sail called Cosmos 1 in a Volna rocket launched from a submarine. But the rocket failed and the spacecraft never reached orbit. Five years later, on 21 May 2010, JAXA launched the world’s first interplanetary solar sail IKAROS to Venus to demonstrate solar sail flight, together with the AKATSUKI probe.
On November 19, 2010, NASA launched NanoSail-D2 with FASTSAT aboard a Minotaur IV, becoming NASA’s first solar sail deployed in low earth orbit. NanoSail-D1 was lost on a Falcon 1 failed launch. On 20 May 2015, the Planetary Society launched a second solar sail called “LightSail-1” to demonstrate satellite systems for the following LightSail-2. Deployment happened in low earth orbit. Following up, on 25 June 2019, LightSail-2 was launched as a secondary payload from a Falcon Heavy launch, the sail was deployed at a much higher orbit and was able to demonstrate orbital maneuvering using the sail.
Worked Example(s)
To date, only a handful of solar sails have actually been worked on, built and actually flown with the intention of testing solar sail propulsion. Here are a few.
Cosmos 1
Cosmos 1 was a joint project between Cosmos Studios and The Planetary Society, to test a solar sail in space. An uncrewed spacecraft was launched on June 21 2005 aboard a small Volna rocket launched from a Russian submarine. But the launch failed to reach orbit. The spacecraft itself weighed around 100 kg and consisted of eight triangular blades connected to a central hub. The Rigging system consisted of inflatable structural tubes. Total surface area of the sail was about and was made of PET film coated in aluminum. The mission was expected to last 30 days, during which the spacecraft would use its sails to boost its orbit by up to 100 km. Had it gone as planned, Cosmos 1 would be the first spacecraft to use a solar sail as propulsion.
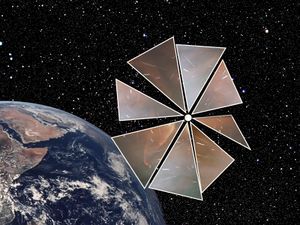
LightSail 1 and 2
The LightSail project was a follow up on the failed Cosmos 1 by The Planetary Society. Two spacecraft were launched, LightSail 1 as an engineering demonstration to test the sail deployment system in orbit. And LightSail 2, to demonstrate using solar sails as propulsion. Each spacecraft was built around a 3U cubesat bus, about 30cm x 10 cm x 10 cm in their stowed configuration. And capable of deploying a Mylar solar sail with an area of 32 m2. The sail was rigged with four 4-meter long booms made of lightweight cobalt alloy.
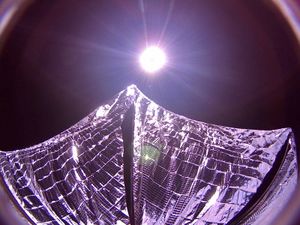
LightSail 1 was launched on 20 May 2015 as a secondary payload on a Atlas V launch. Successfully deploying its sail on 7 June 2015, and reentering the atmosphere one week later. LightSail 2 was launched as a secondary payload on a Falcon Heavy launch on 25 June 2019. Deployed its sail almost a month later on 23 July 2019. And it seems to have demonstrated some limited maneuvering using the solar sail.
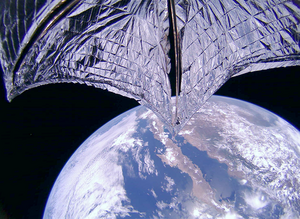
IKARUS
IKAROS (Interplanetary Kite-craft Accelerated by Radiation Of the Sun) is an experimental spacecraft built by JAXA and launched on 20 May 2020, aboard a H-IIA rocket together with the Akatsuki mission, and four other small spacecraft. IKARUS was the first spacecraft to demonstrate solar sail propulsion in interplanetary space. It flew just just over 80 thousand kilometers from Venus. And by 2013, it had changed the spacecraft’s speed by over 400 meters per second.

IKAROS sail was made of metalized polyimide film, with integrated LCD panels to adjust reflectance for attitude control. It also had thin film solar cells built into the sail. The sail was deployed by spinning the spacecraft and releasing four 500 gram tip masses connected to lines on the diagonals of the sail, which then stretched the sail by centrifugal force.
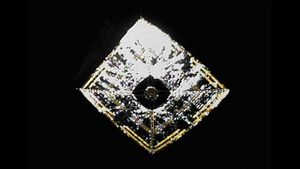
NanoSail-D and D2
NanoSail-D was a small spacecraft built by NASA's Ames Research Center to study solar sail deployment in space. Built around a 3U CubeSat and weighing about 4 kg. It launched aboard a Falcon 1 rocket on 3 August 2008. But the launch failed, and the payload never reached orbit. NanoSail-D2 was originally built as the ground spare for NanoSail-D. After the failed launch in 2008, the spare received several improvements and launched on a Minotaur IV rocket on 20 November 2010. The spacecraft was deployed on 19 January 2011, after the ejection mechanism failed, but ultimately released the spacecraft.
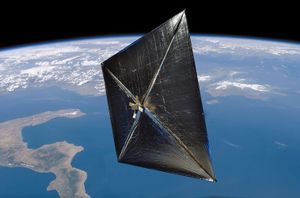
Additional Reading
Additional References
Solar Sails: A Novel Approach to Interplanetary travel - Giovanni Vulpetti Les Johnson Gregory L. Matloff
Solar Sailing: Technology, Dynamis and Mission Applications - Colin R. Mclnnes
- Kim Eric Drexler
The Heliogyro, an interplanetary flying machine
- ↑ K. Eric Drexler, Design of a High Performance Solar Sail System, (New York: Massachusetts Institute of Technology, 1979).